E4: Collective excitations for fun and profit
B123 Birge - (510) 642-7269
Collective excitations are an emergent phenomena, modes of a many-body system with a well defined momentum and energy that can look unlike the constituent particles. However, they depend on---and can probe---the underlying symmetries and physics of the system. A state with broken symmetry is usually associated with a gapless mode known as a Goldstone boson. Gapped excitations are often created by subtle interactions between particles. Well understood excitations in turn provide tools to probe their environment, whether that is phase slips in a superfluid or the inertial environment of the experiment.
The ferromagnetic condensate breaks a total of three symmetries at low temperature. Those broken symmetries lead to two Nambu-Goldstone bosons, phonons with linear dispersion relation, and magnons with quadratic dispersion relation. In addition, the order parameter of the spinor condensate admits a variety of global---topological---structures. In our lab, we're studying these emergent phenomena and exploring ways in which collective excitations can be used to perform unbiased measurements of the condensate and the world in which it lives.
Here are a few examples of interesting studies enabled by collective excitations. For more details, see our ResearchHighlights.
Several phonon modes of the ring BEC
Imaging magnons and phonons
In order to study collective excitations, it helps to be able to image them. We have developed several novel methods of imaging the gas magnetization and density with high signal to noise and a variable level of non-destructivity. These methods allow us to image phonons, as in our work on phonon interferometry (right), as well as magnons and interesting spin textures (below).
Magnon interferometry
Precisely measuring the frequency of a collective excitation allows us to test the underlying physics of a many-body system. Here, we study magnons, collective spin waves, in a spin-1 ferromagnetic superfluid. We create collective excitations of different momenta, which appear as a spin density wave. The contrast of the standing wave oscillates in time as the momentum components evolve, giving a direct interferometric readout of the dispersion relation.
Probing the magnetic dipole interaction with nearly-free magnons
In three dimensions, a spin wave is gapless by Goldstone's Theorem: a global rotation of the spin costs no energy, so long wavelength excitations have a vanishing energy gap. In our two-dimensional system, dipolar interactions introduce a preferred direction and break this symmetry. In the absence of this symmetry breaking, the dispersion of the magnons is unaffected by variations in condensate density. However, in the presence of such symmetry breaking, the shift in spin precession frequency with density and spin direction directly yields the gap.
Magnons as a probe of the superfluid phase
The magnons have the phase of the condensate from which they are created. As counterpropagating magnons flow, the interference pattern shows superfluid phase. Here, we track the motion of a single vortex over four seconds by repeatedly creating and imaging magnons in the same condensate.
Using magnons to measure the lowest entropy atomic gas ever
Measuring temperature can be very challenging using commom methods such as time-of-flight at very low temperatures compared to the condensation temperature. The non-condensed fraction, which carries the entropy of the degenerate gas, can be vanishingly small. However, incoherent magnons within the gas can still have a large non-condensed fraction, and readily reveal the temperature of the gas in their momentum distribution.
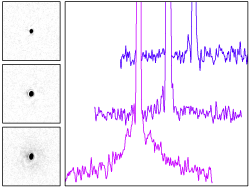 | 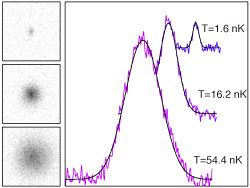 |
Momentum distribution of the condensed atoms at various temperatures. | The temperature is easily determined from the momentum distribution of the magnons. |
Magnons give us the ability to accurately measure temperatures in a previously unexplored regime of low entropy, and have revealed that evaporative cooling, a staple of cold atom physics, can be used to create gases that are far colder than previously imagined, as low as 0.02 times the critical temperature in our experiments.
Temperature of gas after single cycle of decoherence with varying numbers of magnons (points) and repeated cycles (triangles).
Magnons for cooling in a deep trap
Magnons enable two types of cooling that work without lowering the trap depth, which might be constrained, for example, because it also controls the tunneling rate in a lattice: cycled-decoherence cooling/demagnetization cooling and magnon-assisted evaporation. In magnon-assisted evaporation, magnons increase cooling power of evaporative cooling by increasing the non-condensed phase space density. In cycled-decoherence cooling/demagnetization cooling, magnons created in a degenerate gas absorb energy from the majority-spin gas as they thermalize. After thermalization, the magnons can be expelled and the process repeated.
Shot-noise limited rotation noise in a phonon gyroscope
A standing sound wave in a BEC remains stationary as the world rotates underneath it. In the lab frame, a rotation appears as the precession of the standing wave (left). The signal is expected to be insensitive to changes in atom number and trap geometry.
We examine the precession of several acoustic mode standing waves and extract a rotation signal, as well as the properties of the confining trap. The noise (below) in the rotation signal is close to the atom shot noise result.